Preliminary Checks
Measuring the Open Circuit Potential
After connecting the electrodes to the potentiostat using the appropriate leads (consult the user manual to determine which lead corresponds to which electrode), the researcher should measure the open circuit potential using the appropriate command on the potentiostat software. The open circuit potential, or “rest potential,” is the potential of the working electrode when it is placed in solution, and it is determined by the characteristics of the species in solution. The open circuit potential should remain constant if it is measured multiple times; some potentiostats have an option to monitor the open circuit potential over a few minutes. If the measured value is extreme or varies wildly with time, there is most likely a connection problem such as wires are in contact, a bubble on the vycor frit in the reference electrode compartment, the electrolyte concentration is too low, or the vycor frit on the reference electrode is damaged.
Compensating for Solution Resistance
If an acceptable open circuit potential is measured, one can proceed to measure the solution resistance. The potentiostat software should be able to perform this measurement with the click of a button. Sometimes the user needs to input the potential at which the solution resistance is measured, and it is good practice to use the open circuit potential for this value. Checking the solution resistance may also indicate whether there is a problem with the working or counter electrode connections; a resistance of 0 implies a short circuit, whereas a resistance higher than 10,000 Ω suggests that an electrode is not connected, an electrode is not in the electrolyte solution, or there is not enough electrolyte salt in solution. Certain low polarity solvents may give high resistances even at electrolyte salt concentrations of 0.1 M, but this resistance can be lowered by increasing the electrolyte salt concentration.
Solution resistance is, as its name suggests, the intrinsic electrical resistance of the solution between the working electrode and the reference electrode. As in most real-world circuits, each component through which current flows has a resistance associated with it. The electrolyte solution is no different; charge flows in the form of electrolyte ions in solution physically moving towards one electrode or the other, and their motion is impeded by solvent molecules and other molecules in solution. At high currents, uncompensated solution resistance will cause the actual potential of the working electrode potential during the sweep to be different from the potential that the potentiostat thinks it is applying, which will result in broad features in the CV. This is due to the fact that the resistance leads to a change in potential according to the equation V = IR, and V (the difference between the intended potential of the working electrode and the actual potential felt by the working electrode) can get quite large at large resistances (R) and currents (I).
Modern potentiostats can “compensate” for large solution resistances, but as tempting as it is, one should never fully compensate for the solution resistance (0 Ω uncompensated resistance). Solution resistance is actually necessary for proper collection of the CV, but it should be minimized (2 – 40 Ω is usually acceptable). One way to minimize the solution resistance, thereby limiting the amount of compensating required by the potentiostat, is to place the tip of the reference electrode as close as possible to the working electrode. With lower polarity solvents, such as THF, DME, or tetraglyme, a higher concentration of electrolyte salt may help lower the solution resistance also.
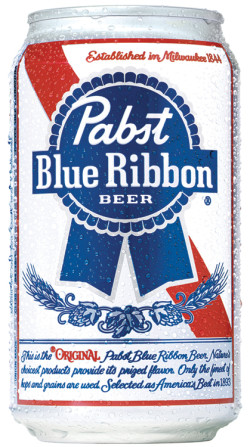
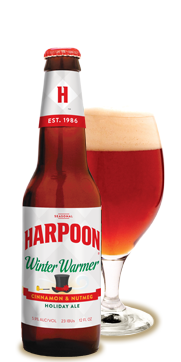
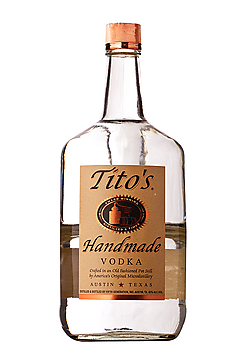
Did I earn one of these yet?

is licensed under a Creative Commons Attribution-NonCommercial-ShareAlike 4.0 International License.